Angle-resolved photoelectron
spectroscopy (ARPES) probes the electronic structure (energy and
momentum) of materials by measuring the energy and angle of the
emitted electrons. In particular, ARPES can determine in "momentum
space" the Fermi surface which represents the locus of the momenta
of the highest energy occupied electron states (Fermi energy). The
Fermi surface is important because electrons near the Fermi surface
are responsible for many physical properties, including superconductivity.
From high-resolution measurements along the Fermi surface in HTSCs,
ARPES has revealed several major departures from the behavior of
conventional superconductors.
Model
showing one orientation of charge stripes in the Cu-O planes. Up
and down arrows represent local magnetic moments in the antiferromagnetic
insulator that separates the stripes. Red circles in stripes represent
holes.
For their ARPES experiments, the researchers studied a compound
known to have stripes, (La1.28Nd0.6Sr0.12)CuO4, whose "parent" compound,
La2CuO4, is an insulator. Copper and some of the oxygen atoms are
arranged on a square lattice in parallel planes with little interplanar
interaction. Replacing some of the lanthanum in the insulator with
strontium (strontium doping), which has one less electron for bonding,
to form (La2-xSrx)CuO4 results in the generation of positively charged
holes (missing electrons) that end up in the copper-oxygen planes.
Over a strontium concentration range (x) from around 6 to 27 percent,
the material becomes superconducting, except at 12 percent where
the superconductivity is suppressed.
|
The High-Temperature Superconductivity Puzzle
|
Stripes, in which holes are confined to parallel lines of copper
atoms in the copper-oxygen planes separated by insulating regions
without holes, were first observed at this so-called one-eighth
doping, suggesting a perhaps antagonistic, but in any case intimate,
relationship between superconductivity and stripe formation. The
replacement of some lanthanum with neodymium stabilizes the stripes
at low temperature. Stripes were later seen at other dopings and
in other superconductors.
The ARPES spectra obtained for (La1.28Nd0.6Sr0.12)CuO4 exhibited several
unusual features. The Fermi surface implied by the data is highly one
dimensional. It has a cross-like shape consisting of two sets of parallel
lines that intersect at right angles. This pattern deviates significantly
from that calculated for the two-dimensional copper-oxygen planes but
is consistent with the superposition of Fermi surfaces from stripes with
two perpendicular orientations. The one dimensionality seems to imply
that the electrons are well confined in the stripes and move along them.
However, the data also indicate that electrons very close to the Fermi
energy show two-dimensional behavior, i.e., electrons can also move perpendicular
to the stripes. The unusual behavior of the stripes may represent a new
state of matter and apparently a new theory is called for to understand
this behavior.
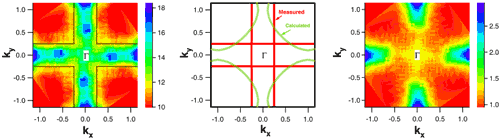
Integrating
the photoemission intensity over a 500-meV range of energies (left)
is a way to visualize the Fermi surface, the boundary between high
(blue) and low (red) intensities. The one-dimensional Fermi surface
determined in this way differs significantly from that calculated
for the two-dimensional Cu-O planes (center). However, reduced intensity
at low momenta (right) when integrating over a narrower range (100
meV) reveals an anisotropic energy gap associated with two-dimensional
behavior.
These results provide new grist for the HTSC mill, whose implications
may reach farther than even superconductivity. The several families of
HTSCs constitute one segment of a still larger class of so-called strongly
correlated materials that are characterized by a powerful Coulomb repulsion
between neighboring electrons. Many physicists believe that solution of
the HTSC problem will require a new paradigm for strongly correlated materials.
Research conducted by X.J. Zhou (Berkely Lab and Stanford Univ.); P.
Bogdanov, S.A. Kellar, and Z.-X. Shen (Stanford Univ.); Z. Hussain (Berkeley
Lab); T. Noda, H. Eisaki, and S. Uchida (Univ. of Tokyo).
Research Funding: Division of Materials Science (DMS), U. S. Department
of Energy; National Science Foundation. Operation of the ALS is supported
by DMS.
Publication about this research: X.J. Zhou, P. Bogdanov, S.A. Kellar,
T. Noda, H. Eisaki, S. Uchida, Z. Hussain, and Z.-X. Shen, "One-Dimensional
Electronic Structure and Suppression of d-Wave Node State in (La1.28Nd0.6Sr0.12)CuO4,"
Science 286(5438), 268-272 (1999).
ALSNews Vol.
144, January 19, 2000 |